With the progress of science and technology and the development of agricultural modernization, the smart tractor will become an important tool for future agricultural production, the emergence of smart tractors so that the tractor from the traditional farming tools into an intelligent farm hub, become the core of sustainable and efficient agricultural practices, but also become the farmers can significantly improve their productivity indispensable existence.
As the “power heart” of modern agriculture, the tractor is basically defined as a traction machine equipped with an electronic engine or a fuel engine, and its core mission is to drive farming equipment such as plowing, planting, and fertilizing to complete field operations through power output. However, under the wave of smart agriculture revolution, smart tractor has broken through the single function of “plowing tool” and evolved into a “farm intelligence hub” that integrates data collection, analysis, and execution by equipping with self-driving module and AI decision-making algorithm.
Traditional agriculture relies on empirical decision-making, but climate change and resource scarcity are forcing an intelligent transformation. Tractors have evolved from “traction tools” to data-driven agricultural hubs, and this paper reveals how they can be utilized in precision agriculture through technological iterations (e.g., autopilot, AI decision-making), and provides a scalable upgrade path and cost-benefit analysis for enterprises and farmers.
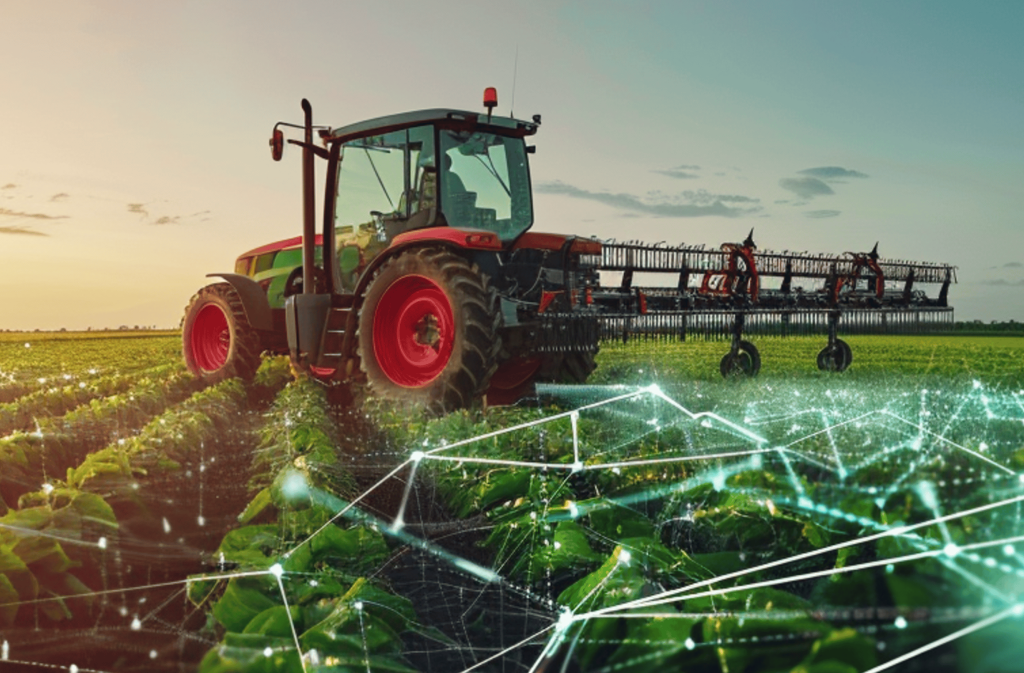
Contents
- 1 I. Limitations of Traditional Tractors: Resource Waste and Efficiency Bottleneck
- 2 Ⅱ. The era of precision agriculture: three major technological leaps for tractors
- 3 Ⅲ. The long-term benefits of investing in smart tractors: Calculate your “economic accounts”
- 4 Ⅳ. How to choose the right smart tractor? 4-step decision-making method
- 5 V. Future Outlook: Tractors will become the “core of agro-ecology”
- 6 VI. Advantages and disadvantages of smart agriculture
- 7 Conclusion
I. Limitations of Traditional Tractors: Resource Waste and Efficiency Bottleneck
In the non-intelligent era, the value of tractor is limited to “power output”, but its rough mode of operation leads to a lot of problems:
Over-reliance on human experience:
In traditional operations, drivers need to adjust parameters such as row spacing and sowing depth based on their experience, which is prone to underutilization of land due to human error.
Definition of Micro Tractor:
Usually refers to small equipment with a power of less than 25 hp (about 18.4 kW), suitable for small farm or horticultural operations. Although this type of equipment is flexible, the traditional version still relies on manual operation, making it difficult to avoid problems such as uneven sowing or repetitive paths.
Waste of resources:
Fuel consumption is high, and traditional tractors are fuel inefficient due to imprecise path planning and repetitive land grinding. Research shows that about 20% of fuel consumption in traditional operations originates from ineffective path coverage.
Excessive fertilizers and pesticides:
According to statistics, about 30% of fertilizers are lost due to uneven distribution, while pesticide overspray is not only wasteful but also pollutes the environment. For example, the actual utilization rate of pesticides is less than 40% in some farmlands in China’s North China Plain due to traditional spraying techniques.
Economic Impact:
The global annual increase in agricultural costs due to the waste of traditional farm machinery resources exceeds 12 billion dollars (FAO data).
Many management blind spots:
There is a lack of real-time monitoring technology to identify pests and diseases or changes in soil moisture in a timely manner. For example, Brazilian sugarcane plantations have suffered losses of more than 30% in a single season due to failure to detect pests in a timely manner.
Technology Comparison:
Traditional farm machinery can only judge crop status through visual observation, while intelligent systems can detect pests at an early stage through multispectral imaging, increasing response speed by more than 70%.
Environmental costs:
Lagging management leads to excessive pesticide use, further exacerbating soil degradation and groundwater pollution.
Case:
-In the Indian state of Punjab, traditional microtractors cause about 15 percent of water wastage annually due to inefficient irrigation caused by operational errors.
-A soybean farmer in Iowa, USA, once suffered a direct loss of more than 20,000 dollars due to an error in the calibration of the planter, which led to a lack of seedling emergence on 10 percent of the land.
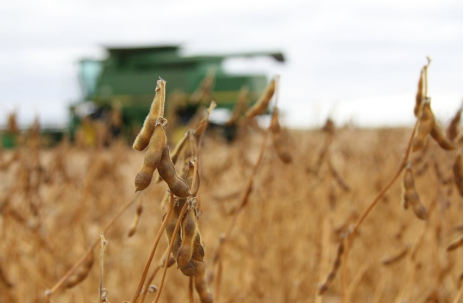
Ⅱ. The era of precision agriculture: three major technological leaps for tractors
1. From “mechanization” to “automation”: the first step to liberate manpower
Automatic driving system:
Based on GPS/RTK positioning technology, it realizes centimeter-level path planning, reduces repetitive grinding, and lowers fuel consumption by 15 percent. For example, Case New Holland’s “Auto-Guide” system can control operating accuracy to within ±2.5 centimeters.
Extended value: Autonomous driving reduces driver fatigue and lowers accident rates (e.g., 40% reduction in accidents on farms in Hokkaido, Japan).
Automatic adjustment of operating parameters:
Dynamically adjusts engine power according to the slope of the terrain, extending equipment life. For example, in hilly areas, the intelligent system automatically reduces power output on steep slopes to minimize engine wear.
Sensor fusion: Real-time monitoring of the machine’s attitude through gyroscope and inclination sensor ensures consistent plowing depth with less than 5% error.
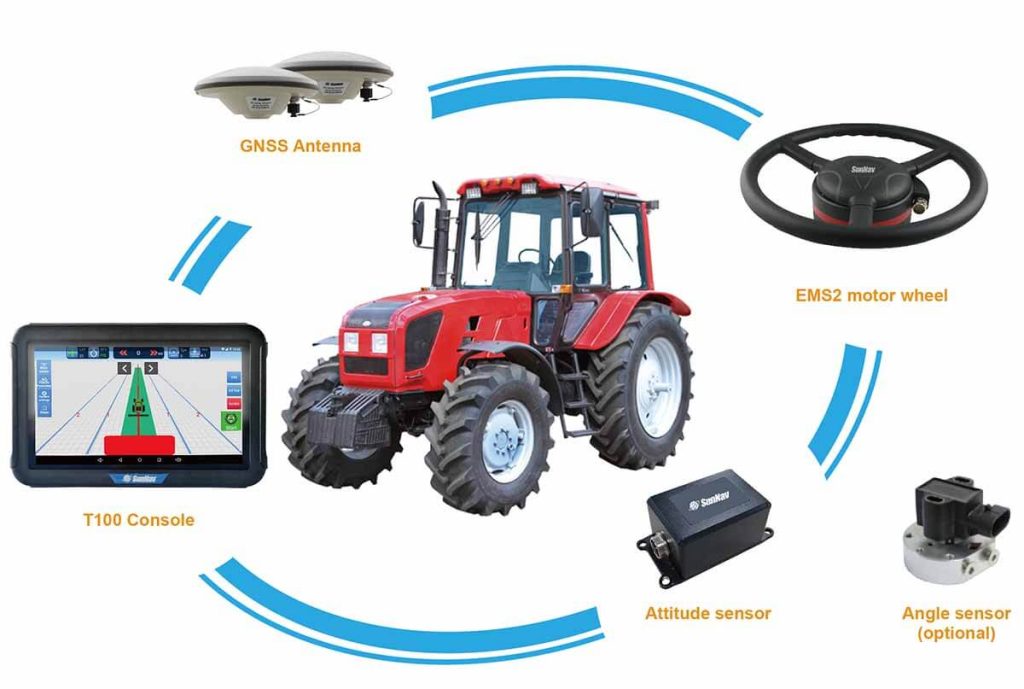
2. From “single-machine operation” to “IoT collaboration”: a data-driven farm network
Multi-machine interconnection:
Tractors share data in real time with seeders and fertilizer applicators to dynamically adjust operating parameters. For example, when the seeder detects abnormal seed flow, it automatically notifies the tractor to slow down and match.
Protocol standard: ISO 11783 (ISOBUS) protocol is adopted to realize the interoperability of different brands of agricultural machines and avoid equipment islands.
Cloud management:
CLAAS’ TELEMATICS system monitors data from 500,000 farm machines worldwide to warn of failures and optimize maintenance cycles. For example, by analyzing engine temperature trends, wear parts can be replaced in advance.
Remote control: farmers can start and stop equipment remotely via a mobile app and view the progress of operations in real time, for example, interrupting field operations in time during the rainy season.
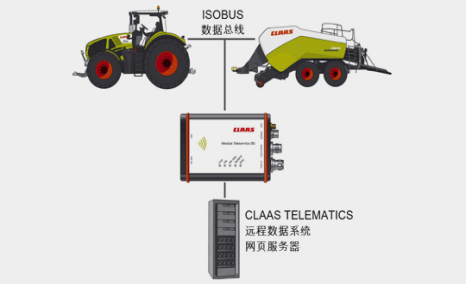
3. From “executing instructions” to “intelligent decision-making”: the farm brain empowered by AI
AI Visual Recognition:
The front camera recognizes the density of weeds and triggers the precision spraying module, reducing pesticide waste (some cases show a 10%-40% reduction in pesticide use). For example, AGCO’s “WeedSeeker” system can distinguish between crops and weeds, and the precision of targeted spraying reaches 90%.
Deep learning applications: Train models with historical data to predict the probability of pest and disease outbreaks. For example, the AI model of Wageningen University in the Netherlands can warn of potato late blight 14 days in advance.
Soil-climate linkage:
Combine with meteorological satellite data to adjust the depth of cultivation, for example, in rice fields, the intelligent decision-making system can improve the mu yield by 12% (need to be combined with specific technical verification).
Dynamic Fertilizer Application: Based on soil nitrogen, phosphorus and potassium content and future rainfall prediction, the system generates variable fertilizer prescription maps, which can increase fertilizer utilization by 25% (e.g., the case of Trimble Ag Software).
Data comparison: Tractors with intelligent decision-making systems can lead to a significant increase in rice yields and a significant reduction in pesticide use.
Ⅲ. The long-term benefits of investing in smart tractors: Calculate your “economic accounts”
1. Cost optimization: visible savings
Fuel costs: 10%-18% fuel savings with autopilot. For example, for a 200 HP tractor with 1,000 hours of annual operation, the annual fuel cost savings can reach 5,000 USD.
Labor cost: 1 smart tractor can replace 3 drivers, saving over 100,000 USD per year in high labor cost areas in Europe and the US (e.g. 18-25 USD per hour for farm workers in California).
Maintenance cost: IoT early warning system reduces sudden failures and maintenance cost by 30% (CLAAS user research data).
2. Risk Control: The Invisible Value
Accurate disaster warning: soil moisture sensors prevent droughts and floods in advance. For example, Israeli drip irrigation farms reduced drought losses from 15% to less than 3% through smart systems.
Compliance guarantee: automatic data recording to meet environmental subsidy applications, and the EU’s “Green New Deal” requires transparent data on carbon emissions from agricultural machinery.
Insurance discounts: Some insurance companies offer premium discounts for smart farm machinery (e.g., State Farm in the U.S. reduces premiums by 12% for farm machinery equipped with self-driving equipment).
3. Sustainability Premium: Seizing the market lead
Green certification: Under the EU’s “carbon tariff” policy, crops produced by low-carbon farm machinery are more likely to enter the high-end market. For example, Bordeaux wine estates in France pay a 20% premium for their products due to the use of electric tractors.
Land preservation: Reducing over-farming and maintaining soil fertility. Sugar cane growers in Queensland, Australia, have seen an 8% increase in soil organic matter content in five years after adopting CNH smart tractors.
Brand value: blockchain traceability data enhances consumer trust, e.g. Japan’s Koshihikari rice increased its export price by 35% by uploading the whole farm machinery data on the chain.
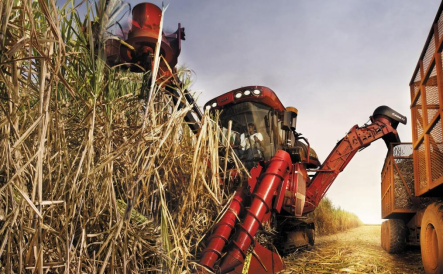
Ⅳ. How to choose the right smart tractor? 4-step decision-making method
1. Demand diagnosis:
Dry fields need high traction models (>500 mu choose 180-220 hp), paddy fields need anti-sag design (such as rubber tracked chassis);
Economic crops (such as vineyards) can choose narrow-body models (width <1.5 meters) to avoid damage to the vine frame.
Power matching formula: Theoretical required power (kW) = Operating resistance (kN) × Operating speed (m/s) ÷ Efficiency coefficient (0.7-0.8).
2. Technical compatibility:
Ensure that the device supports mainstream IoT protocols (e.g. ISO 11783) to avoid information silos;
Verify the openness of the API interface to facilitate access to third-party management platforms;
Data security: choose a brand that supports local data storage or encrypted transmission (e.g. Kubota’s blockchain storage solution).
3. Service network:
Prioritize brands with local 4S service points;
Examine the parts inventory cycle (ideally, key parts are available within 72 hours);
Training support: quality brands provide free operational training (e.g. CLAAS “Academy” program).
4. Return on investment calculation:
Combined with government subsidies to calculate the payback cycle, for example, the payback cycle of small smart models in China is about 3-5 years;
Calculation formula: Payback cycle (years) = (equipment price – subsidy) ÷ (annual cost savings + annual revenue increase);
Sensitivity analysis: Consider the impact of oil price fluctuations (±20%) on fuel efficiency gains and assess the risk threshold.
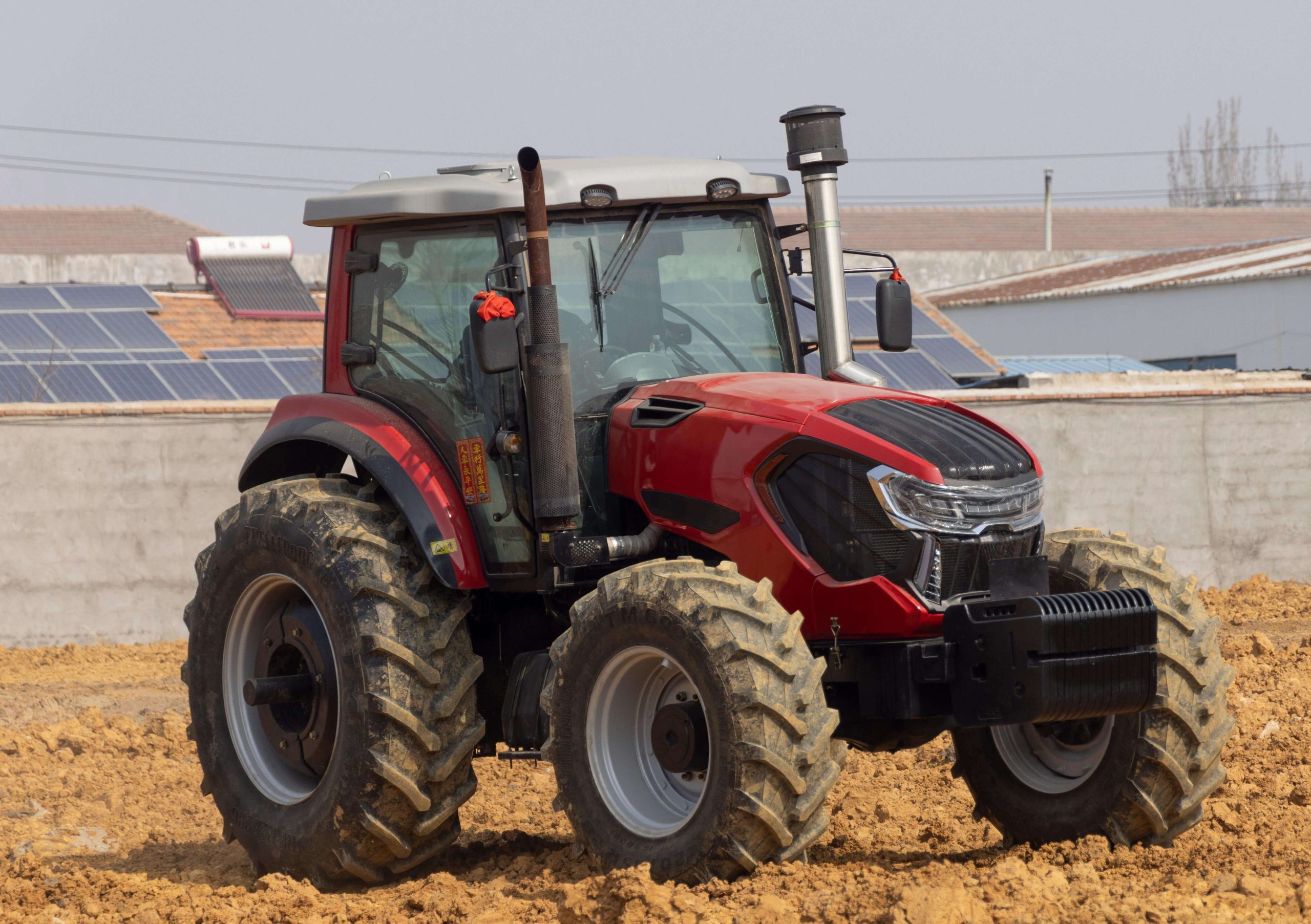
Tool recommendation: with “Tractor Selection Comparison Chart”
Model | Engine Power (kW) | Transmission | PTO Speed (r/min) | Hydraulic Output | Dimensions (L×W×H) mm | Image URL |
LX904 Series | 66.2 | 12F+12R Shuttle | 540/760 | 2-way outputs | 4365×1853×2897 | LX904 Series Image |
High Horsepower 180~220hp | 132.5–162 | 16F+16R | 760/1000, 760/850 | 3-way valve | 5000×2750×3200 | High Horsepower Tractor Image |
50~80 hp 4 Wheel Tractor | 36.8–66.2 | 8F+2R or 8F+8R | 540/760 | 1 or 2-way valve | Varies by model | 50~80 hp Tractor Image |
Small Wheeled Tractor 25~45 hp | 18.4–33.1 | 8F+2R | 540/760 | Simple or forced | Compact dimensions | Small Wheeled Tractor Image |
V. Future Outlook: Tractors will become the “core of agro-ecology”
1. Hydrogen power:
New Holland NH² prototype tests hydrogen fuel cells for zero carbon emissions, with a range of up to 6 hours and hydrogen refueling in just 10 minutes;
Challenges: Hydrogen storage and transportation infrastructure is not yet widespread, current costs are 40% higher than diesel, and parity is expected to be achieved by 2030;
Policy support: Japan has included hydrogen-powered agricultural machinery in its “green growth strategy”, providing a 50% R&D subsidy.
2. Cluster robotization:
Small unmanned tractor clusters (e.g. SwarBot) are adapted to complex terrains such as mountains and greenhouses through 5G synergy, and operational efficiency is increased by 3 times;
Technological breakthrough: edge computing chips (e.g. NVIDIA Jetson) realize real-time obstacle avoidance with response delay <50 ms;
Application scenario: in the Dutch flower greenhouse, the miniature robot crowd can accurately complete the whole process of seeding, transplanting and harvesting.
3.Blockchain traceability:
Data from sowing to harvesting is uplinked (e.g. IBM Food Trust platform), and consumers can scan the code to check the operation track of agricultural machines and pesticide use records;
Business value: Thailand’s jasmine rice has increased its export unit price by 28% due to full chain traceability;
Technology integration: combined with NFT technology, limited edition agricultural products can be attached with digital artwork, opening up new profit models.
VI. Advantages and disadvantages of smart agriculture
Pros:
1. Efficiency revolution:
Resource Utilization Improvement: pesticide use rate is significantly reduced, food production is significantly increased, and the global food gap can be reduced by 15% (UN estimate);
Human liberation: autopilot + AI decision-making reduces 90% of repetitive labor, easing the aging of the agricultural workforce.
2.Environment-friendly:
Precise application of pesticides reduces groundwater pollution; according to German research, intelligent systems reduce pesticide concentrations in watersheds by 60%;
A clear path to decarbonization: electric/hydrogen farm machinery helps to achieve the goal of carbon neutrality in agriculture by 2050.
3. Data empowerment:
Historical data to train models to optimize planting solutions, e.g. Monsanto’s Climate FieldView platform increases corn yields by 8%;
Market risk prediction: guide crop planting structure adjustment through global commodity price fluctuation data.
Disadvantages:
1. High threshold:
High initial investment: smart tractors are 2-3 times more expensive than traditional models;
Technical complexity: requires farmers to master basic digital skills, making it difficult to promote in developing countries.
2.Dependence risk:
Network interruption may lead to system paralysis, a farm in Australia lost $120,000 in 2023 due to loss of satellite signal;
Data security: sensitive information such as farm tracks and yields may be commercially exploited by third-party platforms.
3. Ethical controversies:
Automation exacerbates rural employment pressures, with the International Labor Organization predicting a 9% reduction in global agricultural jobs by 2030;
Technological monopoly: a few companies control core algorithms (e.g. Deere owns more than 60% of agricultural AI patents), squeezing the bargaining power of small farmers..
Conclusion
Precision agriculture is not a concept, but a revolution in the making. Choosing a smart tractor is not only buying equipment, but also introducing a whole set of “data+algorithm+hardware” intelligent solutions. As an industry leader, MINNUO has 36 years of experience in tractor manufacturing and sales, and has provided customized upgrading services to more than 100 farms around the world. Schedule an expert consultation today to get your “Precision Farming Startup Program”!